Scientists now have created a novel ruthenium-based material exhibiting characteristics of the elusive quantum spin liquid (QSL) state, paving the way for breakthroughs in quantum materials and applications.
In the realm of quantum materials, a new milestone has been achieved with the development of a material that exhibits behaviors akin to the elusive “quantum spin liquid” (QSL) state. Researchers at the University of Birmingham have pioneered a ruthenium-based material demonstrating disordered magnetic properties, a phenomenon predicted but never before realized experimentally. This breakthrough opens the door to new possibilities in quantum science, particularly in understanding and applying quantum states of matter.
Redefining Magnetic Materials
Traditional magnetic materials, like the familiar bar magnets, operate on a principle of ordered magnetism where electrons align to create north and south poles. This alignment underpins classical ferromagnetism, familiar in household and industrial applications. Quantum spin liquids, however, challenge this paradigm. These materials exhibit disordered magnetic states where electrons interact through quantum entanglement rather than alignment. This disordered behavior defies conventional physics and has intrigued scientists for decades.
The Birmingham team’s achievement lies in engineering a material that approaches the “Kitaev quantum spin liquid state,” a theoretical model proposed in 2009 by physicist Alexei Kitaev. Unlike traditional materials, this ruthenium-based compound offers a pathway to studying magnetic interactions at the quantum level, potentially reshaping fields from quantum computing to material science.
Why Quantum Spin Liquids Matter?
Quantum spin liquids represent a fundamental shift in understanding magnetism. They operate on the principles of quantum entanglement, where particles remain interconnected regardless of distance. This characteristic offers immense potential for quantum applications, particularly in developing robust quantum computing systems.
One of the key challenges in realizing QSLs has been the tendency of candidate materials to revert to conventional magnetic order due to tightly packed ion structures. These dense arrangements lead to strong magnetic interactions that override the subtle quantum effects theorized in QSL states. The Birmingham team’s material, with its open framework, circumvents this issue, enabling weaker magnetic interactions and greater experimental flexibility.
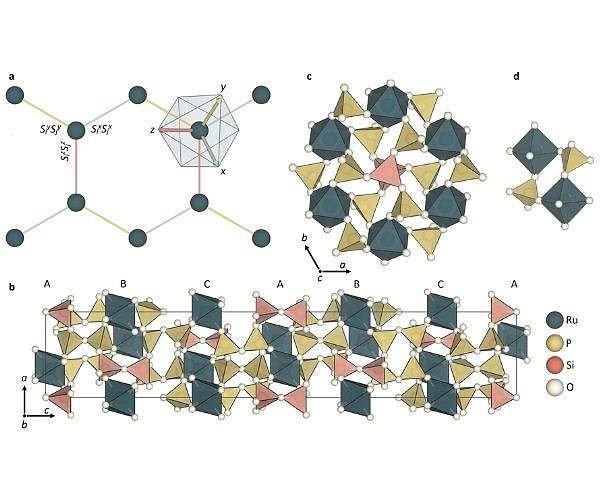
Experimental Leap and Material Design
Using advanced facilities like the ISIS Neutron and Muon Source and the Diamond Light Source in the UK, researchers were able to demonstrate that the ruthenium ions within this new material could be manipulated to produce quantum spin liquid-like behaviors. This open-framework structure provided the flexibility needed to tune the magnetic interactions, an essential step toward realizing Kitaev’s model.
Dr. Lucy Clark, the study’s lead researcher, highlighted the significance of this breakthrough: “This work is an important step in understanding how we can engineer materials to explore quantum states of matter. It opens up a large family of underexplored materials that could yield critical insights into magnetic properties for quantum applications.”
Professor Bruce Gaulin, Director of the Brockhouse Institute for Materials Research at McMaster University, said: “This material appears deceptively simple, with terbium spins decorating a two-dimensional, triangular architecture. But with the full complement of modern experimental techniques at our disposal, the low-temperature magnetism of this structure, based on two distinct terbium environments, exhibits an altogether exotic quantum disordered state of matter – an unexpected and exciting result.”
Challenges and the Road Ahead
Despite this progress, significant challenges remain. Theoretical and practical difficulties have long hampered the study of QSLs. Modeling these materials produces competing magnetic interactions, making predictions notoriously difficult. While the new ruthenium-based material is not a perfect Kitaev material, it bridges the gap between theoretical predictions and experimental realization, offering a platform for future research.
One persistent obstacle is achieving stable QSL states without reverting to magnetic order. The Birmingham team’s solution—creating a more open crystal structure—represents a promising avenue, but perfecting the conditions for Kitaev quantum spin liquids remains elusive. Further experimentation with similar materials may refine these properties, bringing theorists and experimentalists closer to achieving a fully realized QSL.
Implications for Quantum Science and Technology
The ability to engineer quantum materials with disordered magnetic properties has profound implications for several fields:
- Quantum Computing: QSLs’ reliance on entangled electrons could form the basis for fault-tolerant quantum bits (qubits), enhancing the stability and scalability of quantum computing systems.
- Material Science: The discovery expands the toolkit for designing materials with tunable magnetic behaviors, offering applications in advanced sensors and energy-efficient technologies.
- Fundamental Physics: Understanding QSLs could illuminate new aspects of quantum mechanics, pushing the boundaries of what is known about the behavior of matter at subatomic levels.
This discovery underscores the importance of interdisciplinary collaboration in advancing quantum research, combining theoretical insights with state-of-the-art experimental techniques.
A New Frontier?
The development of a ruthenium-based material approximating quantum spin liquid behaviors marks a pivotal moment in the study of quantum materials. While the journey to perfect QSL states is far from over, this breakthrough demonstrates the feasibility of bridging theory and experimentation. By exploring the magnetic properties of these disordered states, researchers are unlocking new realms of possibility, from redefining magnetic materials to laying the groundwork for quantum technologies of the future. The road ahead promises to be as challenging as it is revolutionary, but each step forward reaffirms the transformative potential of quantum science.
Lastly, the creation of a ruthenium-based material approximating quantum spin liquid behaviors marks a pivotal moment in quantum research. It bridges the gap between theory and experimentation, offering a new platform to explore magnetic interactions and quantum entanglement. While challenges remain in achieving stable QSL states, this breakthrough lays the groundwork for future discoveries in quantum computing, material science, and fundamental physics.